
Measuring the waves through Titan: ISAS builds a seismometer for NASA’s Dragonfly mission
In June this year NASA announced the selection of Dragonfly for the agency’s New Frontiers program. The mission will fly an innovative multi-rotor drone through the atmosphere of Titan, investigating multiple sites on the Saturn moon. The goal is to study chemistry similar to that which supported the development of life on the early Earth.
Onboard Dragonfly will be a seismometer designed here at ISAS・JAXA to investigate the interior of one of the most Earth-like locations in the Solar System.
“A multi-rotor vehicle is not instantly an obvious platform for seismology!” notes Ralph Lorenz, Project Scientist for Dragonfly who is based at the Johns Hopkins Applied Physics Laboratory. “But investigating Titan’s habitability requires knowing what is happening under the surface.”
Receiving 1000 times less heat from the Sun per unit area compared with the Earth, the average temperature on the surface of Titan is a staggeringly cold -179°C. Yet rather than a bare ball of ice, Titan is a world with an atmosphere, lakes and rain.
The liquid on Titan is not water but consists predominantly of methane; a simple organic molecule that is a gas on Earth. Titan’s extreme conditions allow methane to evaporate, condense and rain-out in a cycle that mimics the behaviour of water on Earth. But could this Earth-like analogue extend to supporting the chemistry needed for life? Answering this question is the task for Dragonfly.
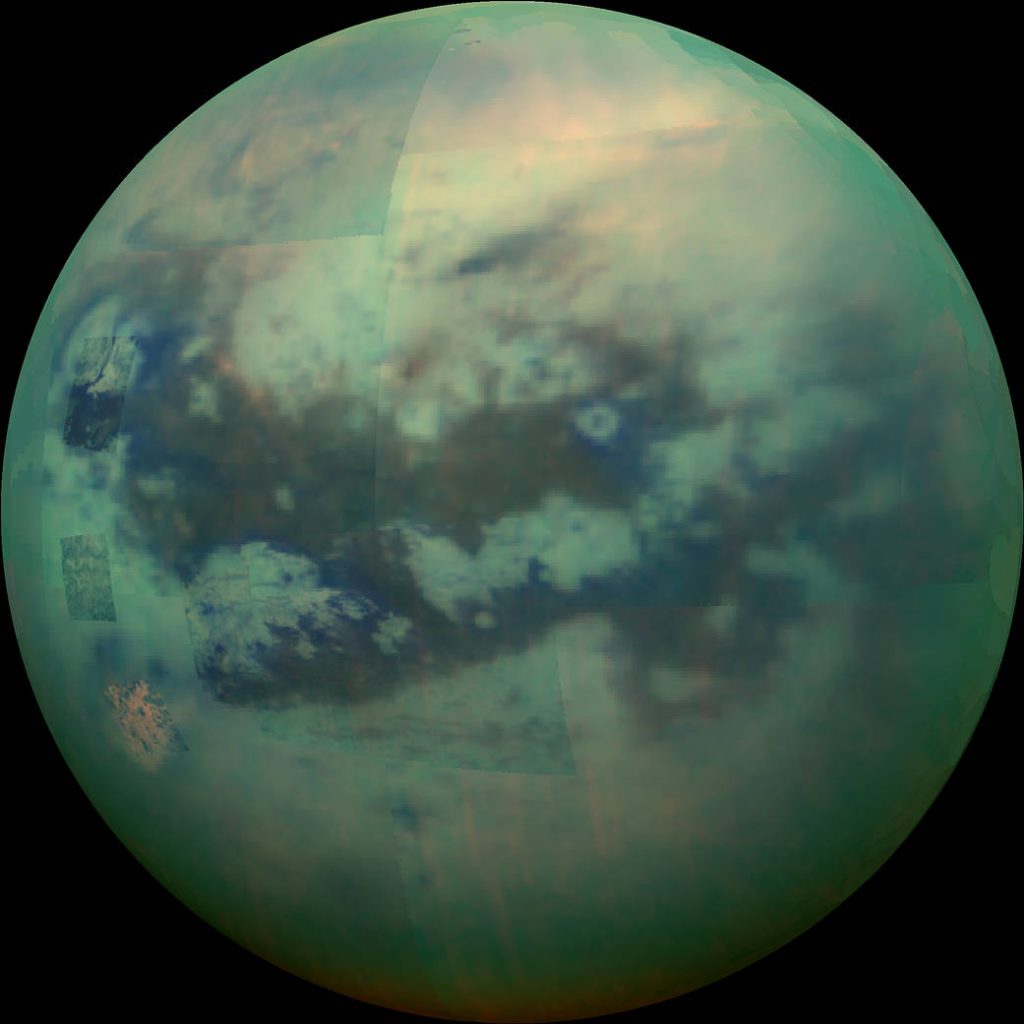
Titan has been visited once before by the NASA Cassini mission to Saturn that carried with it the ESA Huygens probe that was deployed to the surface of Titan. Huygens descended through the Titan atmosphere for 2.5 hours, before successfully landing on the surface and returning data for a further 72 minutes.
Data from the mission confirmed that the surface of Titan is covered by layer of reddish organics over a thick icy crust. These organics likely formed in Titan’s atmosphere as ultraviolet from the Sun split apart methane molecules to create larger organics that sank to the surface as solids.
Beneath the ice crust is thought to be an internal water ocean, similar to the hidden oceans of Europa and Ganymede. What is less clear is the thickness the ice, which could lie anyway between about 50km to 200km in depth. It is an important value, as water is an excellent solvent for building complex organic molecules such as those needed for life. Transport between Titan’s organic-laden surface and the hidden ocean could therefore give rise to prebiotic chemistry that might then be ejected back to the surface and be detectable.
There is evidence that the interior and surface of the moon may be connected. The atmosphere of Titan holds more methane than that contained in the surface lakes, suggesting methane is being added to the atmosphere from another source. Without replenishment, Titan’s methane would have been steadily converted into other organics as it was broken apart by the Sun’s ultraviolet. The most likely explanation is episodic emission of methane from the moon’s interior. But occasional outbursts would not mean the crustal ice is thin enough to allow substantial exchange between the subsurface ocean and surface.
This is where seismology is important. Titan is on a slightly elliptical orbit around Saturn, causing the giant planet to flex the moon as its gravity waxes and wanes across the moon’s body. These tidal stresses create heat that helps keep Titan’s hidden ocean from freezing. Such squeezing will also cause the icy crust to creak, sending seismic vibrations through the moon. The vibrations will pass through the ice and encounter the ocean, reflecting at the ice-water interface.
We regularly observe similar reflections in light waves. Seeing your image in a window, or squinting at the glare from the surface of a lake, is due to light being reflected as it encounters a different medium.
The reflected seismic wave will pass back through the ice and may be reflected again as it hit the surface of the moon. The wave can therefore be detected multiple times as it bounces through the ice crust. The timing between detections can reveal the depth of the ice, as a longer intermission corresponds to a thicker crust that the wave must travel through.
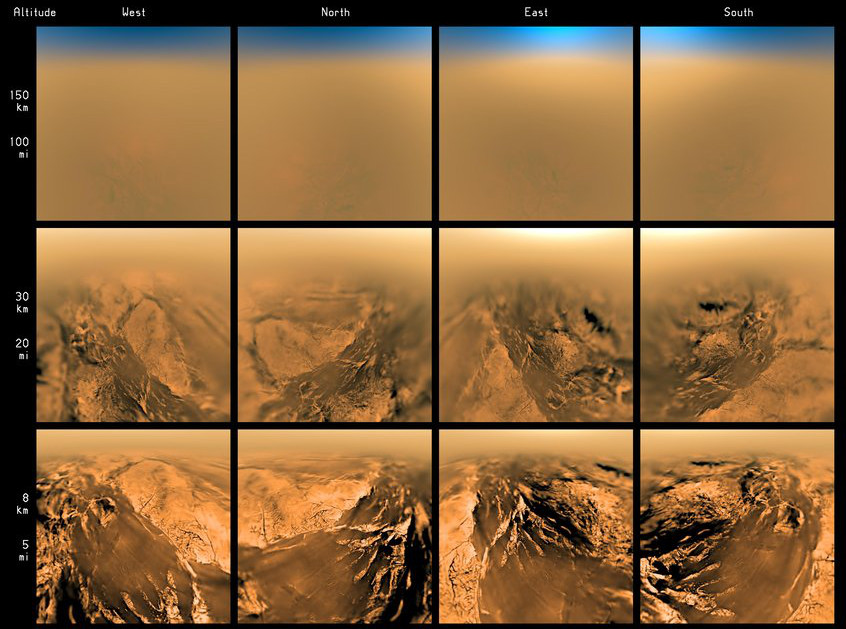
In charge of detecting the Titan creaks is Assistant Professor Hiroaki Shiraishi (白石浩章) in the Department of Solar System Sciences at ISAS. The seismometer he has designed is rugged and compact, making it ideally suited for repeated flights through the harsh conditions on Titan.
A seismometer works by suspending a pendulum above the ground. When the ground shakes due to a quake, the relative motion between the stationary pendulum mass and the moving ground reveals the strength of the seismic shudder. Since a pendulum cannot float unaided, seismometers suspend the pendulum by a mechanism that transmits the motion of the ground as weakly as possible.
In the seismometer that will fly on Titan, a wire coil is wrapped around the pendulum which is held suspended by springs. The case of the seismometer (which remains connected with the ground) has a magnetic interior. As the ground shakes, the magnetic case will move relative to the coil and this induces an electric current (a process known as electromagnetic induction). The strength of the current measures the size of the seismic wave.
The design adopted by Shiraishi for his seismometer is no stranger to harsh conditions. His inspiration came from the seismic sensors that are used to measure activity on the ocean floor. When he was looking for a seismometer that could survive conditions in space, Shiraishi approached the same company that had a history in designing instruments that could withstand the sea bottom.
The seismometer designed by Shiraishi and his team was originally developed for LUNAR-A: a planned mission to measure quakes on our own Moon by embedding seismometers in the Moon’s crust. The requirement that the seismometer had to penetrate the lunar surface meant the design had to be both compact and robust. While LUNAR-A was not pursued, the same design was ideal for a device that needed to survive multiple deployments on Titan’s surface.
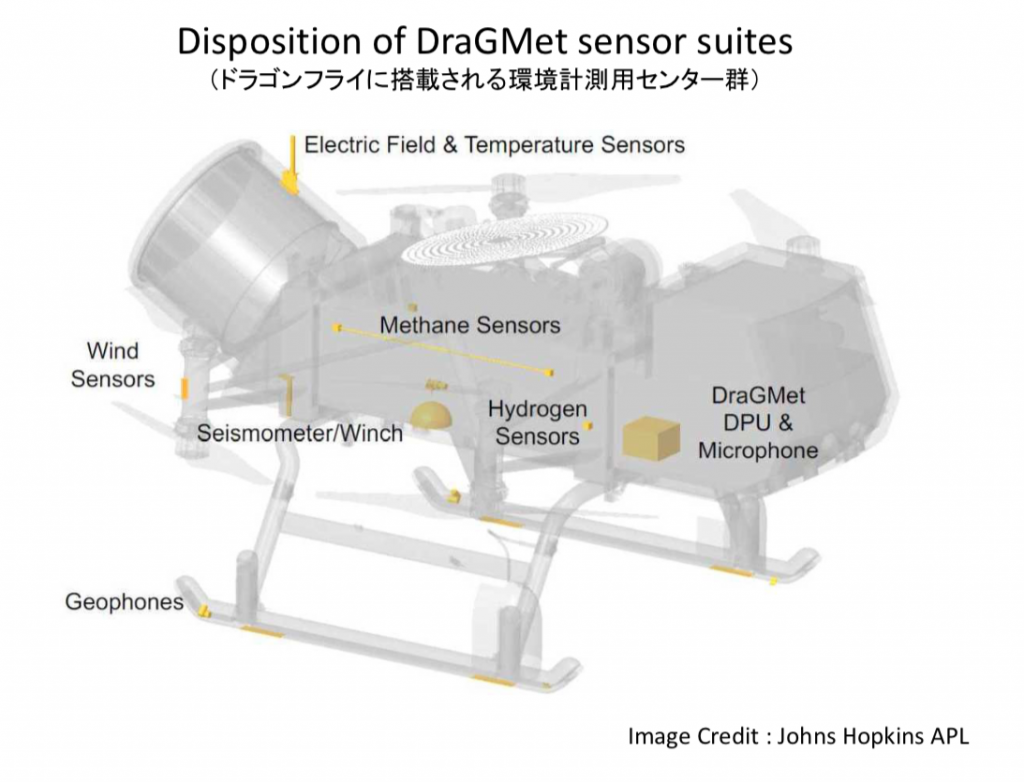
The hardy design also meant that few adjustments were required to make the seismometer suitable for Titan conditions. The main concern was the cold, so Shiraishi’s team checked the performance of the seismometer in a bath of liquid nitrogen. At lower temperatures, springs oscillate more strongly than in temperate conditions. The same motion of the seismometer pendulum on Earth would therefore correspond to a weaker quake on Titan. Characterising the link between the seismic vibration and the motion of the pendulum in Titan conditions is necessary to correctly interpret the recorded data.
Titan will not be the first celestial body where quakes have been measured. Moonquakes have been measured from seismometers placed on the Moon by Apollo astronauts, and at the end of last year, the NASA InSight mission arrived on Mars equipped with a seismometer to investigate the interior of the red planet. As part of a stationary lander, the InSight seismometer required only a single deployment. This allowed for a device substantially heavier than what Dragonfly can afford to carry, and it took several weeks to fully deploy.
“A standard seismometer for terrestrial seismology is about 15kg,” explains Shiraishi. “The InSight seismometer package is 11kg, while our one-component seismometer is only 300g.”
Dragonfly’s seismometer will be deployed and retracted, a process taking just a few minutes, perhaps dozens of times during the mission at different sites.
The lightweight retractable design does come at a cost of sensitivity. The heavier the pendulum within the seismometer, the more sensitive the instrument is to shakes. This added sensitivity is key for InSight, which hopes to detect vibrations on very long timescales, such as the tidal pull from the Martian moons. On Titan, the main sources of seismic activity are expected to produce shorter waves.
A one-component seismometer can also only detect waves in a single direction, rather than in all three spatial dimensions as is common for terrestrial seismology. To counter this, Dragonfly will also be equipped with two simpler geophones that will sit horizontally on the drone’s skids. While far less sensitive than the ISAS seismometer, the geophones will be mounted orthogonally to allow vibrations in all directions to be detected.
The ISAS seismometer will be lowered to sit on the surface of Titan using a winch and will be protected by a windshield. Cameras mounted on Dragonfly will point downwards towards the ground to assess the terrain and ensure the ground is flat enough for the seismometer to rest comfortably.
Even if Titan proves to be seismically silent, seismometer readings can be excited when Dragonfly uses the two drills that will also be installed on the skids. How fast these mechanically generated vibrations can die away can inform on the composition and structure of the Titan regolith.
Dragonfly is set to launch in 2026 and arrive at Titan in 2034. Equipped with eight rotors, the rotorcraft will take full advantage of Titan’s thick atmosphere to fly between sites in a mission that would be impossible on airless bodies such as our Moon or Europa or Ganymede. During the planned 2.5 years of exploration, Dragonfly hopes to explore over 20 sites and cover a total distance of over 150 km. The data the explorer sends back to Earth will inform us about what building blocks of life could form on the moon, from inside to out.