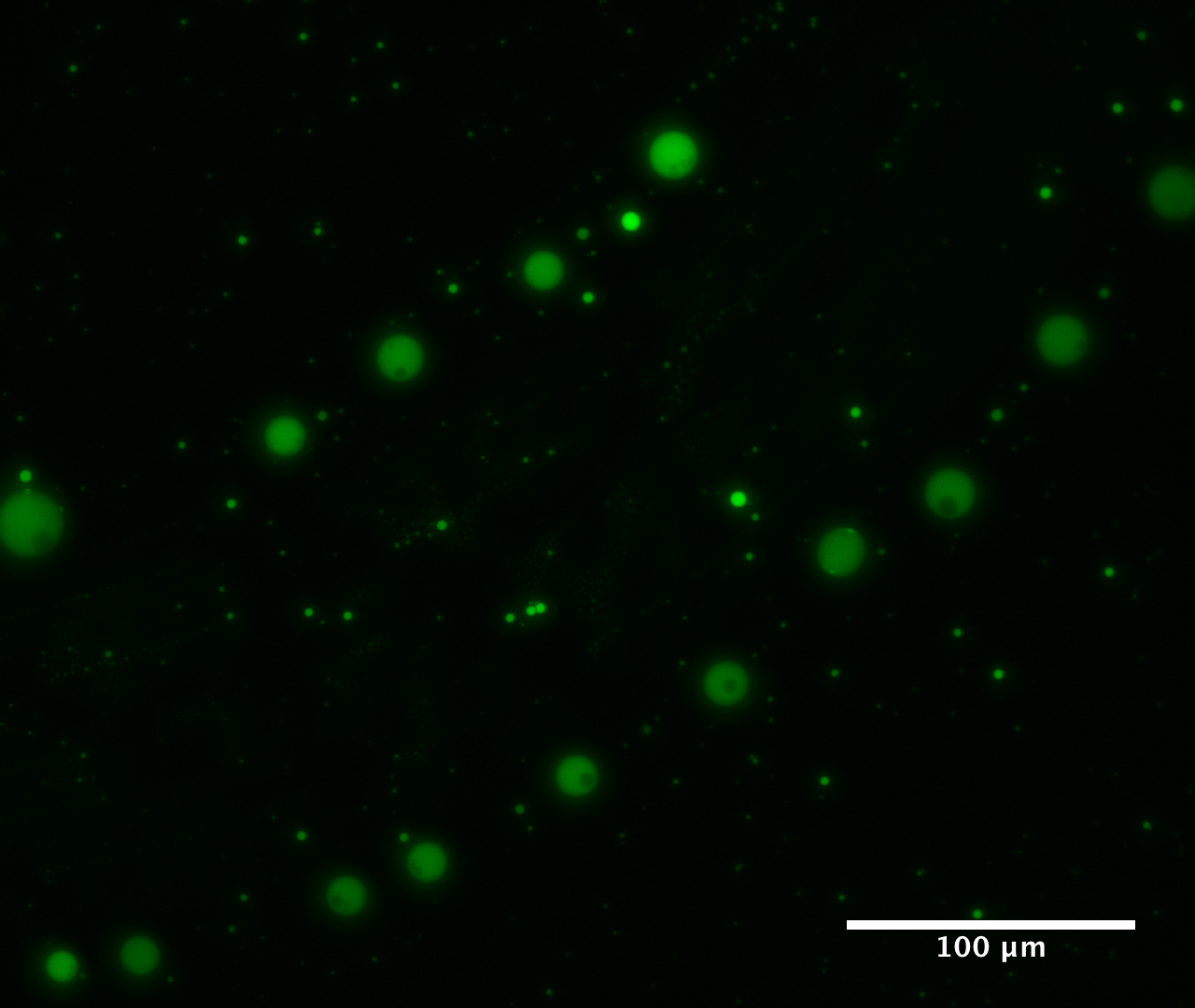
Building the first cells for the origins of life
When the Hayabusa2 mission returns to Earth at the end of 2020, it will bring with it a sample from a carbonaceous asteroid. This class of asteroid is thought to have pelted the early Earth, delivering water and possibly the first organic molecules with which to begin life. But what happened after that?
It is a question that Professor Tomohiro Usui in the Department of Solar System Sciences at ISAS・JAXA is keen to answer.
“How do we go from delivery to something resembling life?” Usui asks. “In my research I’ve been trying to build a pipeline from the movement of the core ingredients for life to something that looks like it could become a biological system.”

Usui is co-author of a recent paper published in the Proceedings of the National Academy of Sciences (PNAS). Led by Assistant Professor Tony Jia at the Earth-Life Science Institute (ELSI) in Tokyo and Assistant Professor Kuhan Chandru from the National University of Malaysia, the team found that carbonaceous asteroids may have also delivered the material needed to make a primitive cell.
Examine any living organism under a microscope and you will see that its body consists of small compartments we call cells. These cells consist of a semi-permeable membrane surrounding material that includes our DNA. The development of the cell is considered a crucial stage in the emergence of life. The tiny compartments provide boundaries for molecules needed in biochemical reactions that would otherwise diffuse away.
Cells today have a membrane consisting of a double row of phospholipids; a complex organic molecule that consists of a head region and two tails. It seems unlikely that life could have begun with something so complicated. But if compartments are needed for significant metabolic action, then another segregating mechanism must have existed.
Previously considered options for early cells have included forming a membrane from fatty acids; simpler molecules with just a single head and tail. Or avoiding building a membrane entirely with substances known as ATPSs (aqueous two-phase systems) that naturally separate out into distinct compartment, rather like oil and water.
However, fatty acid membranes are unstable if there is a high concentration of magnesium cations (magnesium atoms with a negative charge), which are needed to boost the activity of an RNA; an early form of DNA. They are also tricky to form in conditions thought to be present on the early Earth. Meanwhile, the segregation of ATPSs risks breaking down if the level of water changes, such as during rainfall. This leaves the best mechanism for early cells an open question.
Jia, Chandru, Usui and their team turned their attention to chemicals known as α-hydroxyacids, or simply αHAs. αHAs have been found in meteorites, suggesting they may be part of the package that arrived on the early Earth from asteroids or comets and feasibly, may have been delivered to many other rocky worlds in the Galaxy.
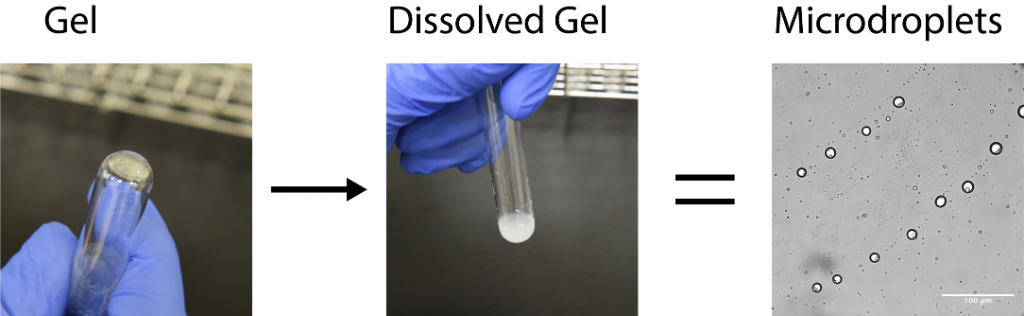
The researchers discovered that when a solution containing αHAs is dried out, the αHAs polymerise into long chains of molecules. Their structure resembled a gel which, when rewetted, forms tiny droplets up to ten micrometers across. These microdroplet compartments looked remarkably like cells.
The microdroplets formed in an acidic solution with a pH value of 2 – 3 (a pH of 7 is a neutral solution, while higher pH values are alkaline). When rewetted to convert the αHAs from a gel into microdroplets, the solution needed to be a mix of water and another solvent called acetonitrile. However, these conditions would not be unexpected on the early Earth. As today, the range of acidic and alkaline conditions would likely have taken a range of values, while acetonitrile is thought to have been a common solvent on the prebiotic Earth.
But while these microdroplets looked the part, could they fulfil the same job as a cell? The team set about testing the droplets functionality at performing cell-like tasks.
The first test was the droplets had to be robust. If they coalesced or dissipated too easily, they could not preserve the chamber long enough to concentrate molecules for chemical reactions. It is also possible that continuous individuality of cells is needed for Darwinian evolution.
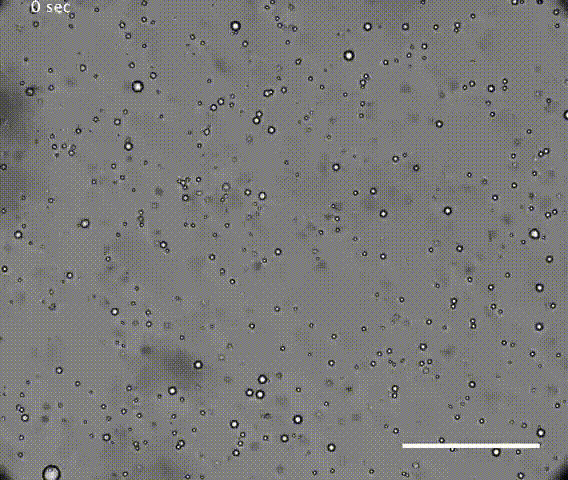
The solution of microdroplets was shown to be stable to increases in the amount of water, suggesting they could survive during rainfall. Coalescence of droplets was quite slow in an acidic solution but the droplets did merge more rapidly in neutral or alkaline conditions. However, merging was suppressed if the solution of microdroplets was agitated, mimicking conditions that might be found on a shoreline or in the hydrothermal vents such as those in Yellowstone. Hydrothermal vents have very high temperatures, but the resilient droplets were not destroyed at temperatures even as high 90°C.
The second test for cell-like behaviour was whether other molecules would enter the droplets and remain there, allowing the compartments to build concentrations of chemicals and facilitate reactions. The team tested this using different types of fluorescent dyes and also fluorescently labelled RNA (RNA with a fluorescent molecule added to the structure) whose location could be easily spotted. Both the dyes and RNA were able to successfully enter and remained in the droplet.
Then there was the question about whether being inside a microdroplet would prevent a molecule from reacting normally. If sitting within the droplet affected the function of the biomolecule, then the benefits of concentration would be undermined.
To test this, the team chose a molecule called superfold green fluorescent protein (sfGFP) that only fluoresces when correctly folded. If the protein is forced into a three-dimensional structure different from its native shape, then it cannot function and does not fluoresce. However, the sfGFP did fluoresce within the microdroplets, promisingly indicating that at least some proteins were able to preserve their usual structure within the droplet environment.
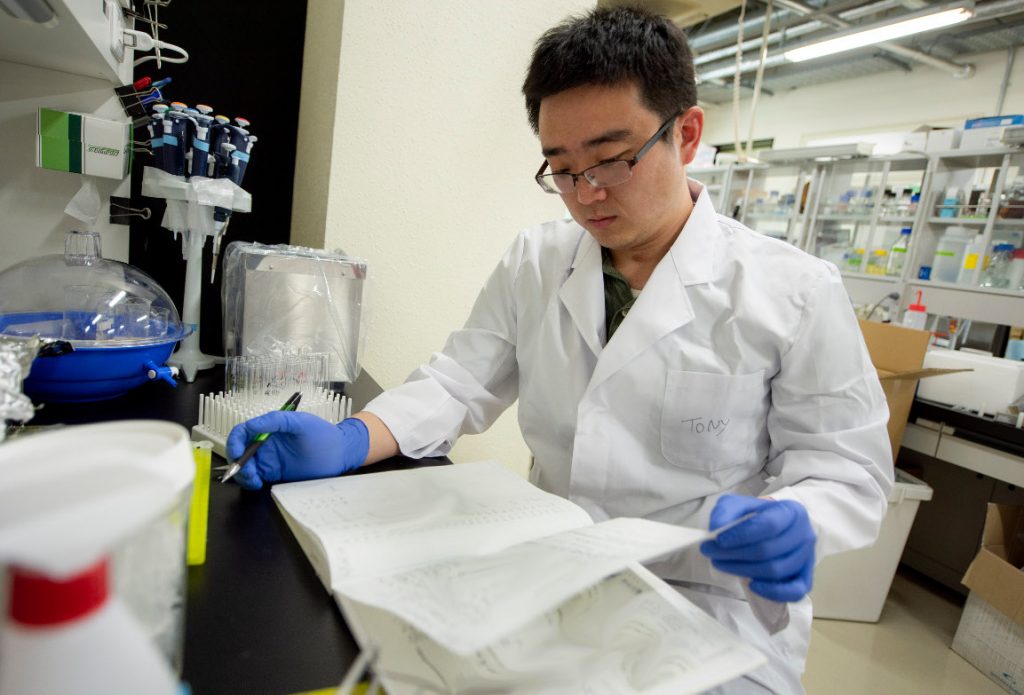
Intriguingly, material entering the microdroplets were not the only interesting results. The team noticed that one dye they tried localised strongly to the outside of the droplet. The molecules in this dye were amphiphilic, meaning they consisted of both a water-loving part (structures that wanted to be near water molecules) and a water-hating part. This split personality is also true for the phospholipids molecules that make up cell membranes today. This means it is possible that microdroplets might not just concentrate chemicals needed for early biological reactions, but may also have acted as a scaffold for the precursors of our own cells to form.
“We don’t know if microdroplets are direct ancestors of cells,” explains Jia. “But they can concentrate materials and perform this scaffolding. Whatever conditions exist on the early Earth, I think microdroplets would have been compatible and could have been relevant.”
This flexibility allows microdroplets to potentially play a key role in the production of all primitive chemistries, not just the ones directly linked to modern biology. Their formation and behaviour could therefore influence how different local conditions arise both on the Earth and other planets as well.
The discovery that microdroplets might have been able to form on the early Earth was the product of a very interdisciplinary collaboration, bringing together expertise on the scale of planets down reactions in a test tube. Talking about how the project began, Usui says he had not previously thought his research would enter a prebiotic chemistry and biochemistry laboratory until he talked with Jia and Chandru at Tokyo’s interdisciplinary institute, ELSI.
“I was having a beer with Tony (Jia) and Kuhan (Chandru) in the ELSI lounge,” Usui explains. “Until then, I was totally unaware that a planetary scientist like myself would have any skills useful to a biochemist!”
The beginning of life is a story of everyone on Earth and it is clearly one that will need all our expertise to piece together.