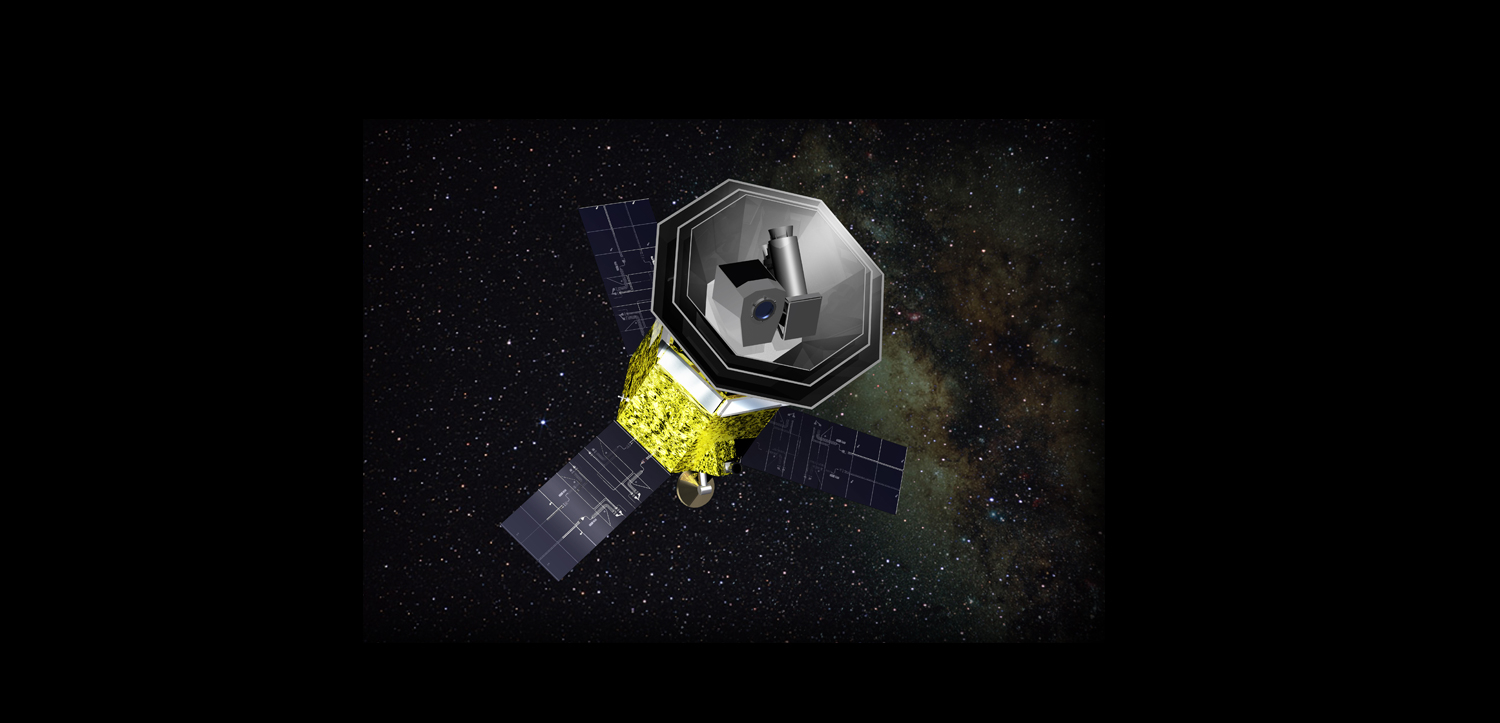
LiteBIRD aims to find evidence for the greatest expansion in the history of the Universe
Approximately 13.8 billion years ago, the Universe is believed to have exploded into existence in an event known as the Big Bang. But what happened immediately afterwards has been difficult to determine. Searching for evidence is the task proposed for the ISAS mission LiteBIRD, which is currently scheduled to launch in 2027.
The earliest information we can gather from the Universe comes from its oldest photons. These particles of light are from the moment when atoms first formed. Prior to this time, the Universe was so exceedingly hot and dense that electrons and protons could not bind to one another and existed as a charged soup. Photons in this era were scattered continuously by the charged particles, moving only a tiny distance before being reorientated. The effect would be like waving a strong magnet over a computer hard-drive, reorienting its internal magnets and continuously resetting its memory.
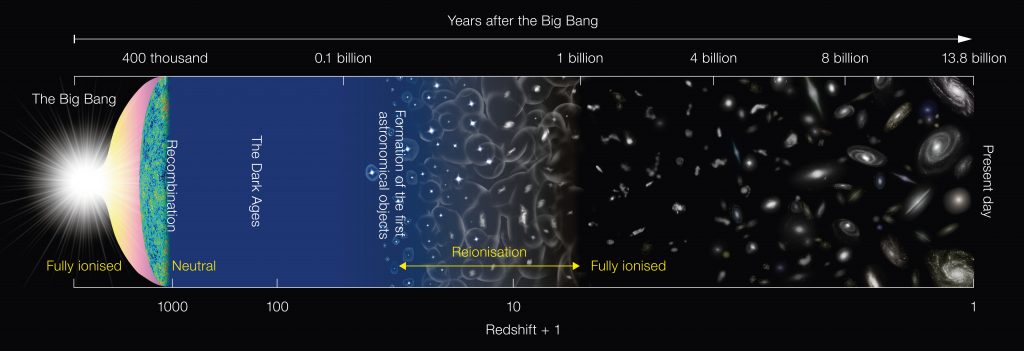
But as the Universe expanded and cooled, atoms were able to form. Neutral atoms do not interact with photons as easily, allowing the photons that existed in that moment to avoid being scattered again. Billions of years later, detectors built on Earth would see these photons as the “cosmic microwave background” (CMB). Their properties depend on the conditions when the photons were last scattered; a snapshot of the Universe as atoms began to form. It is a time known as “recombination”.
“I feel like the most interesting and fascinating aspects of researching the CMB is its richness of cosmological information and how we can probe the fundamental physics of the earliest moments of the Universe,” says Frederick Takayuki Matsuda, Assistant Professor at ISAS who is part of the optics design development for LiteBIRD.
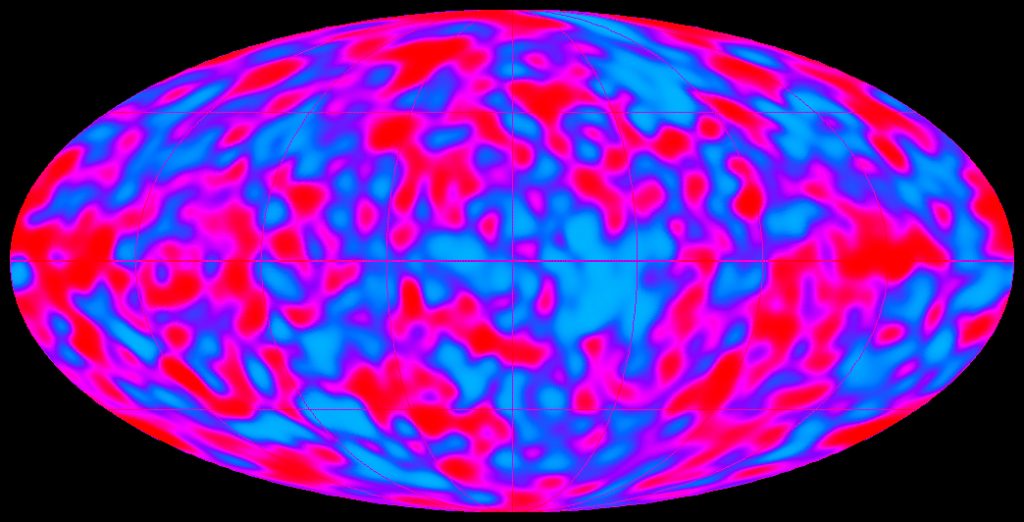
The CMB was first detected in 1964 as an excess signal that appeared to come from all parts of the sky. In 1989, NASA launched the Cosmic Background Explorer (COBE) which revealed these ancient photons were now at a temperature of just 2.725 K (-270°C), cooling as the Universe expanded. This temperature was found to be extremely uniform across the whole sky, but tiny variations of 1 part in 100,000 were detectable. Both these facts would prove to be important.
“COBE discovered there are tiny differences in the CMB temperature depending on where you look on the sky,” explains Oguri Shugo, Assistant Professor at ISAS who is part of the mechanical design development and the detector evaluation for LiteBIRD team. “These are a little like wave patterns on the surface of water. The sizes of the different patterns present have information about the composition of the early Universe.”
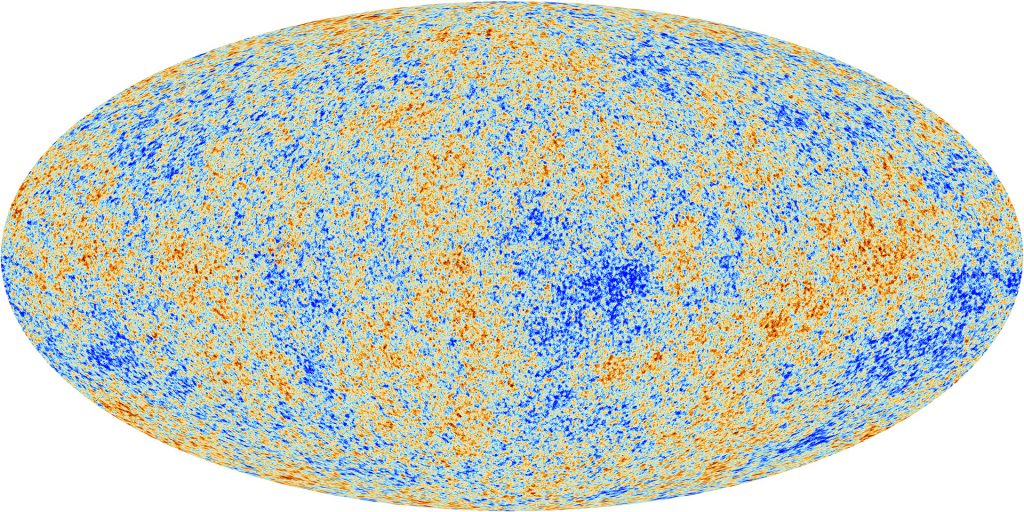
The properties of these tiny waves in the CMB temperature revealed details about the make-up of our Universe. The missions that came after COBE including NASA’s WMAP and ESA’s Planck satellites, found that the CMB suggests that the Universe is spatially flat (two parallel beams of light will stay parallel forever), the density of baryons (regular matter) and the quantity of the elusive dark matter. Yet despite these successes, there are also mysteries.
One of these is the explanation for the incredibly uniform temperature of the CMB, disturbed by only tiny anisotropies. To reach such consistency, photons must have been able to mix together across the whole sky. However, a photon that was emitted at the time of recombination only appears to have had enough time to travel across a small part of the sky since the beginning of the Universe.
To put this more scientifically, the CMB is nearly isotropic even at locations that seem to not be causally connected (i.e. cannot ever have been in contact with one another). This is referred to as the “horizon problem”.
So if the CMB photons have not been able to mix and evenly distribute their heat, how are they all miraculously at the same temperature? The answer may lie in another property of the CMB photons that LiteBIRD is aiming to measure. This is the photon polarisation.
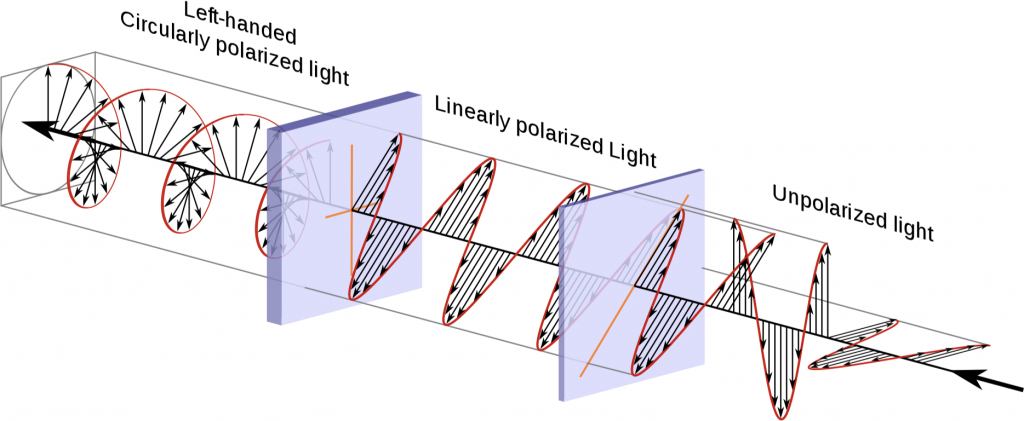
Photons consist of oscillating electric and magnetic waves. The orientation of the wave oscillation is known as the “polarisation”. Light that oscillates in a single plane is said to be linearly polarised. Polarised sunglasses usually block linearly polarised light that oscillates in the plane horizontal to the photon’s direction of motion. This eliminates of much of the light that causes glare, such as reflections from water or the ground.
When temperatures cooled in the early Universe and recombination occurred, the final scattering of photons by the remaining loose charges formed a population of linearly polarised photons. The oscillation plane of this polarisation was strongly affected by the tiny fluctuations in density that would eventually gather material into the stars and galaxies. This forms a pattern of horizontal and vertical plane polarisation across the sky known as the “E-mode” signal. However, theory predicts that there should be another pattern. One that reflects a huge stretch in space.
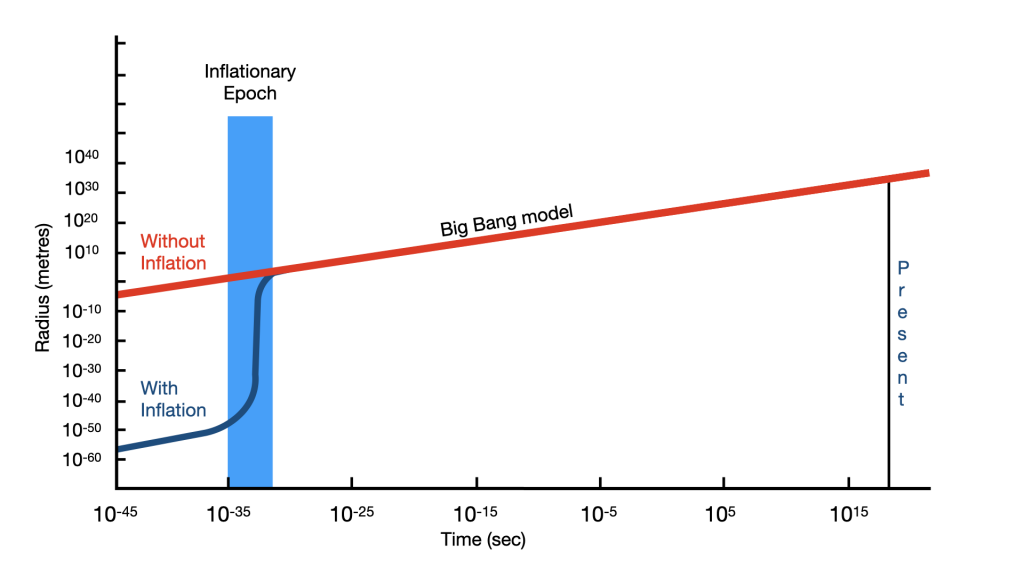
The problem with the CMB photons not having time to mix can be addressed if the Universe underwent a rapid expansion in a tiny fraction of a second after the Big Bang. This would have allowed all regions of the Universe to have been connected to one another in the brief period before inflation occurred.
“Inflation theory claims that quantum-level fluctuations are rapidly stretched to cosmological scales through rapid expansion,” says Oguri. “This expansion strains space, and creates primordial gravitational waves.”
The primordial gravitational waves created during this rapid acceleration of the Universe are now far too weak to directly detect with current experiments. However at recombination, these waves would have been able to affect the direction of the polarisation of the photons, creating a distinct polarisation pattern imprinted on the sky. This is known as the primordial “B-mode” and it is this imprint that LiteBIRD aims to detect.
“Primordial B-modes, if detected, will be ‘smoking-gun’ evidence that inflation occurred in the early Universe,” notes Matsuda.
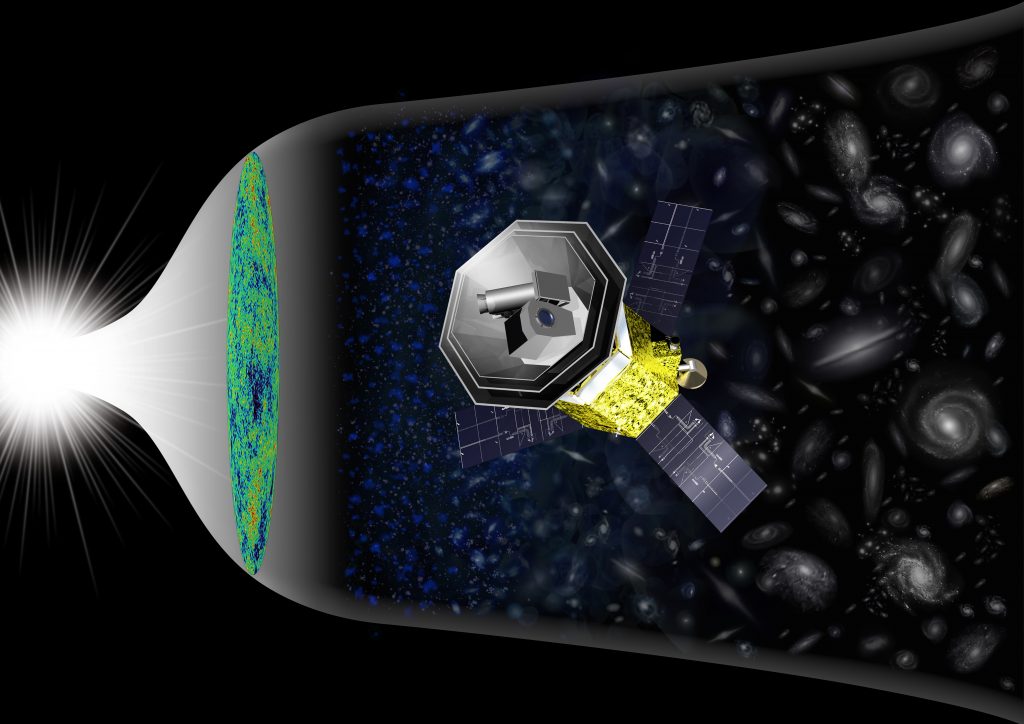
The strength or amplitude of this primordial B-mode smoking gun pattern is unknown. Referred to as the tensor-to-scalar ratio (the amplitude of the B-mode pattern relative to the temperature variation pattern), LiteBIRD aims to measure this value to a precision of less than dr < 0.001 during the three years of the satellite’s nominal mission.
“Although we talk about inflation theory, there are many different variations of this theory,” explains Oguri. “The amplitude of the gravitational waves is not exactly predicted. So if LiteBIRD can measure this, we can identify which theory is correct for our Universe.”
The primordial gravitation wave pattern is predicted to be most easily detectable on scales larger than approximately 1 degree across the sky; twice the width of the full Moon. Previous attempts to measure this primordial signature have so far been unsuccessful, although upper limits on its strength have been found. One of the challenges is that the B-mode polarisation from primordial gravitational waves can be contaminated by the interstellar dust signal. This turned out to be the origin of observations made by the ground-based BICEP2 telescope at the South Pole.
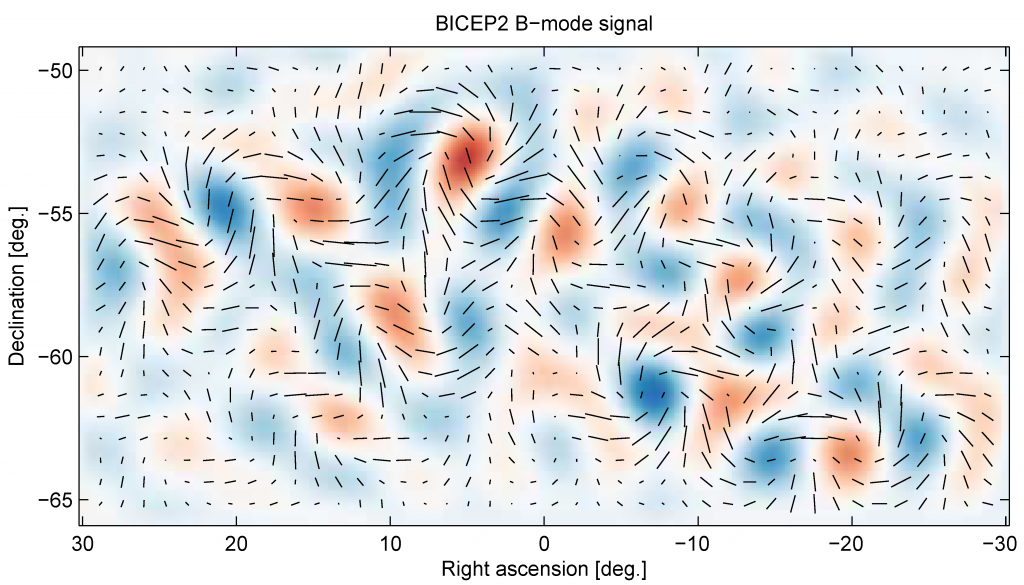
“Even though researchers in the field were already cautious about the existence of foreground contamination, the BICEP2 measurement showed that the dust foregrounds can easily overwhelm the primordial B-mode we’re trying to detect,” Matsuda reflects.
A way to filter out these rogue signals is to measure the sky signal at different frequencies. B-mode signatures caused by contaminates such as dust are expected to vary with frequency differently to the signature produced by inflation. An advantage of LiteBIRD is the satellite will have the ability to conduct a full sky survey at 15 different frequency bands between 34 – 448 GHz. Many of these frequencies are blocked by our atmosphere, making this a job best done from space.
LiteBIRD is a JAXA-led mission with strong international involvement. CNES (France) is constructing one of the satellite’s two onboard telescopes, both of which will also have electronics contributed by CSA (Canada). Additionally, the telescopes’ sensor system is being developed using US technology by QUP (The International Center for Quantum-field Measurement Systems for Studies of the Universe and Particles), and the satellite cooling system is a combined effort from JAXA, NASA, CNES and ESA (Europe).
This wide involvement reflects the importance in these ancient photons in revealing information about the very earliest days of the Universe. They are the light from the first snapshot of how everything began.
Further Information: