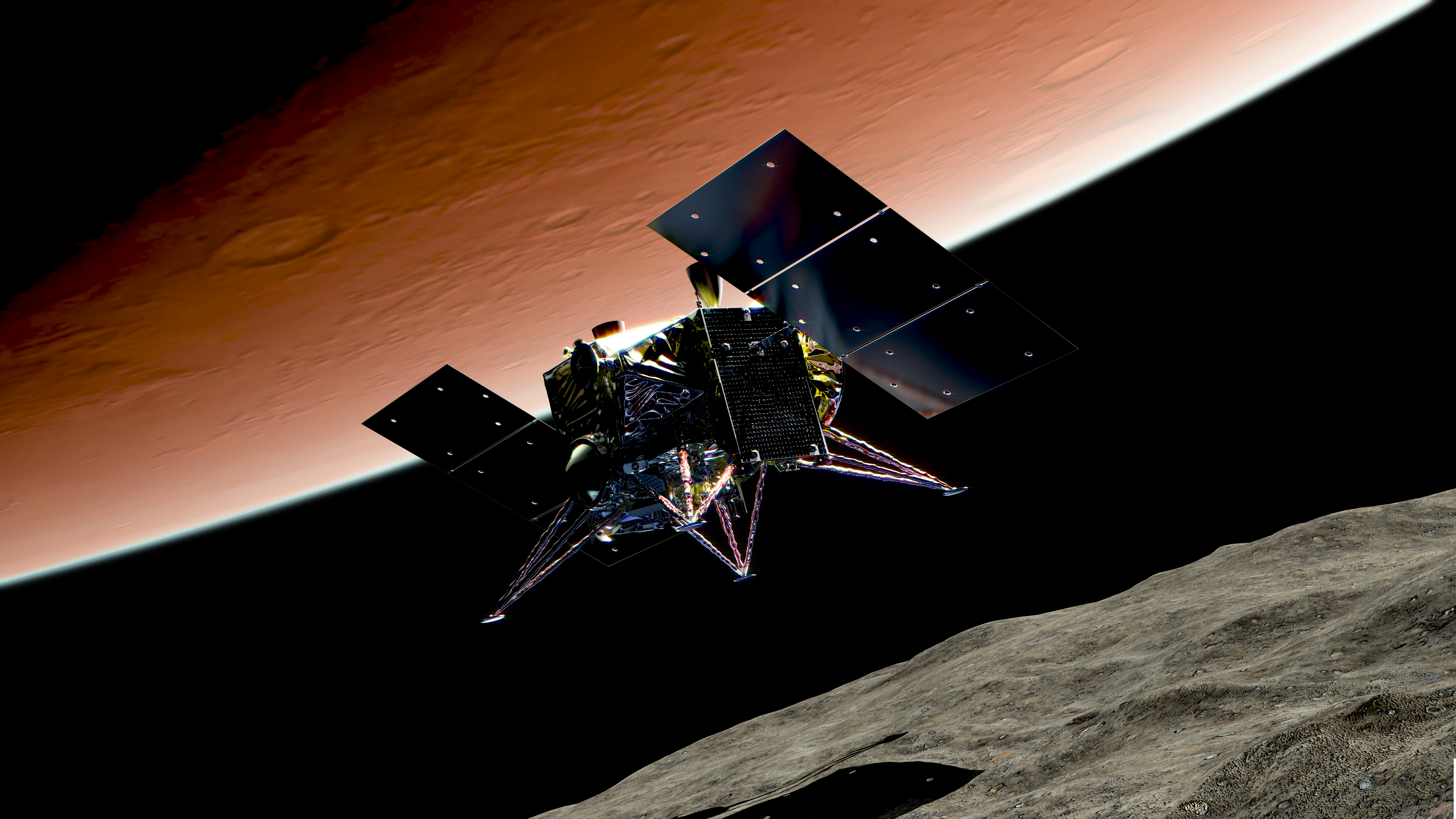
Why explore the moons of Mars?
Dr Ryuki Hyodo shares the science behind JAXA’s upcoming MMX mission to the Martian moons, and the unique features of this journey to Mars’s domain.
In February this year, the world watched in awe as three space missions arrived at Mars in quick succession. The first two were orbiters; the UAE’s Hope mission that will capture a global view of Mars’s climate, and China’s Tianwen-1 with a focus on Martian geology and a planned release of a lander and rover to the Martian surface. The third in the trio was the NASA Perseverance rover, which completed a stunning touchdown in Mars’s Jezero Crater, where it will search for evidence of past life and gather samples for future return to Earth.
At ISAS, researchers watched the progress with particularly keen attention. In just a few years from now, we are about to attempt the same feat of visiting the Martian sphere. But for us, the destination is not the red planet but its two small moons. The Martian Moons eXploration (MMX) mission is scheduled to launch in the fiscal year of 2024. Largely ignoring the looming presence of Mars, the spacecraft will focus its suite of observing instruments on the moons, Phobos and Deimos. The mission plans to land on Phobos and collect samples to bring back to Earth in 2029. It is these barren moons that scientists believe contain evidence of the early days of the Solar System, and how habitability may have flourished and died on the planet below.
Dr Ryuki Hyodo is researcher in the division of Solar System Sciences at ISAS, working on simulations of how the moons formed. Hyodo holds one of the institutes independent ITYF (International Top Young Fellowship) positions; a program designed to support and promote talented researchers from around the world in the early stage of their career. He explains that the first mystery surrounding Phobos and Deimos is how they came to be there at all. In fact, there are two main competing theories for how the moons formed.
“There’s the capture origin, whereby a passing small object is gravitationally captured by Mars,” Hyodo explains. “This was historically proposed and is supported by the spectral similarities of the moons to D-type asteroids.”
Asteroids largely reside in the appropriately named ‘asteroid belt’ that orbits the Sun between Mars and Jupiter. Within this population, asteroids can be divided into different types based on similarities in the wavelengths of the light that reflect off their surface. This ‘spectrum’ is related to the asteroid’s composition. D-type asteroids are notable for their very dark colour. What little light is reflected by the D-types is at longer red and infrared wavelengths.
While many different asteroids now orbit in the asteroid belt, their differing compositions point to formation locations spread across the early Solar System. This is interesting to scientists trying to map the creation and movement of resources, especially those such as water and organics that are needed for life.
If Phobos and Deimos are examples of D-type asteroids that swung close to Mars and were pulled into orbit, then a sample from Phobos could tell us about the formation and transportation of the first organic molecules to form in the Solar System. But not everyone believes this formation scenario.
“The second option is the giant impact origin,” says Hyodo. “A large impact with Mars that ejected material to form a debris disc around the planet.”
Such an impact might be the origin of the Borealis basin; the largest depression on Mars that covers a massive 40% of the planet’s surface. Smaller basins, such as the Utopia or Hellas basin, may also have generated enough debris to form the moons.
“The impact velocities are similar even in the case of the smaller basin-forming events,” notes Hyodo. “The difference is just the impact mass. This leads to similar thermodynamic outcomes for impact ejecta.”
Thermodynamics refers to the heat energy in the debris disc, and determines properties such as how much of the disc material is molten and the amount that will vaporise. The resulting material becomes the building blocks of Phobos and Deimos, as it collides and coalesces into the two moons.
The formation within a disc can explain the near-circular orbits of Phobos and Deimos in the same plane around Mars’s equator. A giant impact is also thought to have created our Moon, but the evidence there is clearer, thanks to samples from the lunar surface returned by the Apollo missions.
“In the case of our Moon, the Apollo sample has strongly indicated that the Moon was once molten and that the Moon and Earth are isotopically very similar,” explains Hyodo.
Isotopes are atoms of the same element that have slightly different weights due to the numbers of neutrons in the atom’s nucleus. Two bodies that consist of not only similar substances, but the same balance of isotopes, are very likely to share common building blocks, supporting an impact scenario where material from the Earth formed the Moon. The energy in a giant impact would also lead to molten material.
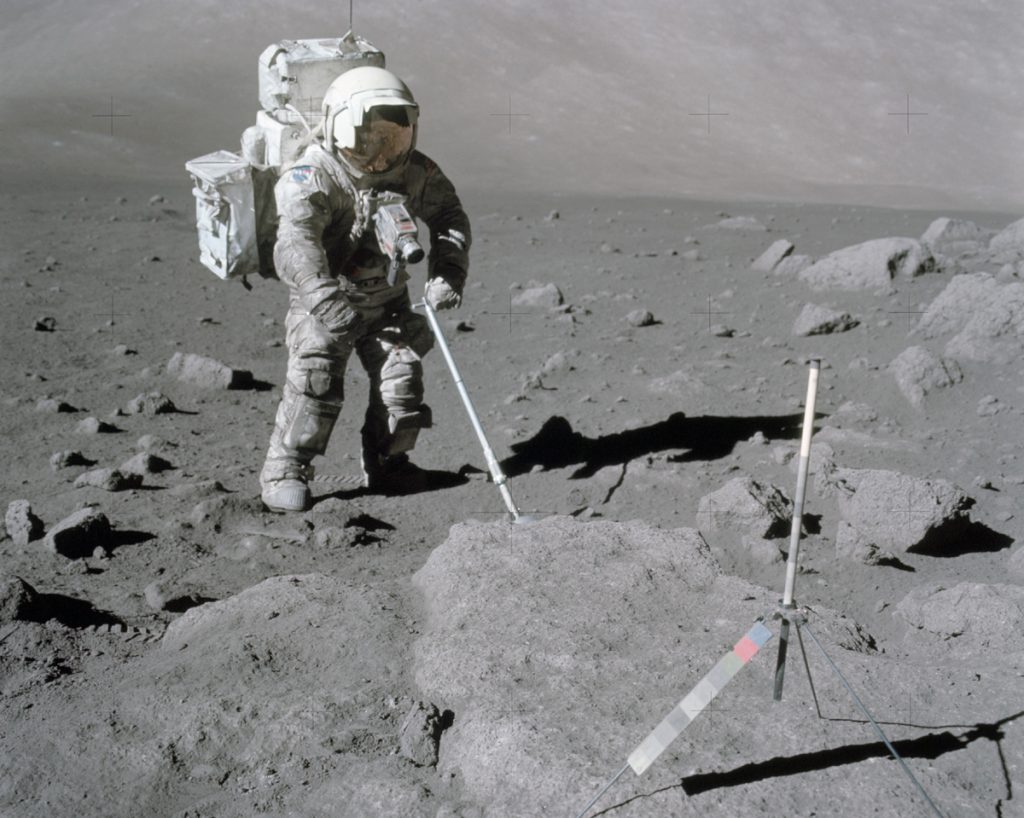
“In the case of the Martian moons, their dynamics (orbits) supports a giant impact formation,” continues Hyodo. “However, without a sample like that from Apollo, we cannot be sure about what happened on Mars and its moons.”
It is not only the initial formation of the moons that is debated but what happened next. A flurry of recent papers have proposed different scenarios for how the moons may have developed after a giant impact.
“It is important to note that these works all assume the impact scenario,” begins Hyodo. “The difference between them is what happens after the giant impact occurs and effects the tidal evolution of Phobos.”
The inner of Mars’s two moons, Phobos is slowly being pulled inwards to the planet’s surface. This is due to Mars’s gravity distorting the moon by raising tidal bulges that result in a drag force that pulls the moon inwards. The end evolution is likely to see Phobos ripped to pieces before it collides with the surface. In one possible scenario, this inevitable death scene for the moon has been replayed during Mars’s history multiple times. The first inner moon to be created during the giant impact quickly spiralled inwards and was shredded by Mars’s gravity. This formed a new debris ring out of which a second generation moon was born. Research suggests as many as five Phobos incarnation may have occurred before the moon we see today.
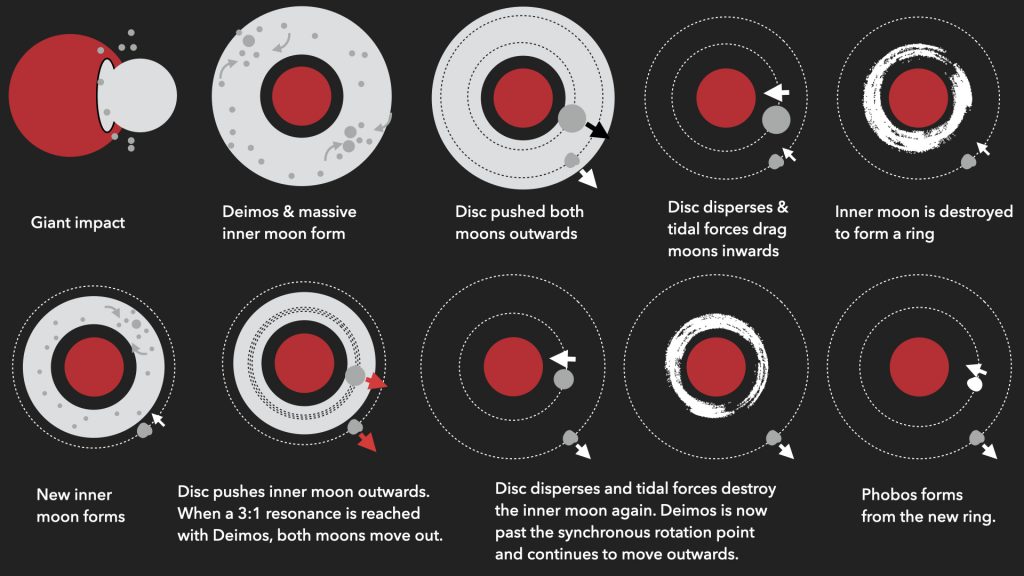
Another idea is that the Phobos and Deimos were once a single body that was itself subject to an impact that split it into two several billion years ago. This suggested scenario is based on the how the moons’ orbits may have changed due to the tides from Mars, and detailed simulations still need to be performed.
“Particle accumulation is a chaotic process,” notes Hyodo as he describes computer simulations of the moons forming within the debris disc of the giant impact. “Sometimes we only form a single moon or sometime three moons. If a single moon was initially formed from a giant impact and later destroyed to split in two, then this story may be possible.”
The sample of Phobos material collected by the MMX spacecraft will provide scientists back on Earth with the opportunity to analyse the moons of Mars in the same way as the history of our own Moon was unpicked from the Apollo samples. This, Hyodo confirms, will help resolve the degeneracy between the theories.
“If the sample includes a large amount of Martian material as well as volatile depletion, the answer is the giant impact origin, not capture,” he claims.
Simulations run by Hyodo confirm that any debris resulting from a giant impact should include about 50% of Martian material, with the rest originating from the impactor. The impact will also produce strong heating (around 2000 Kelvin or 1730°C), so elements that can easily turn to gas (volatiles) will be vaporise and escape.
“The tricky part is the long-term evolution of Phobos,” admits Hyodo. “A detailed measurement of the gravity field of the moon as well as observations to clarify the internal structure will be key to constraining how the tides from Mars’s gravity have been pulling on the moon. Constraining the surface age is also important, as each story suggests a different time for the final accumulation of the Phobos that we know today.”
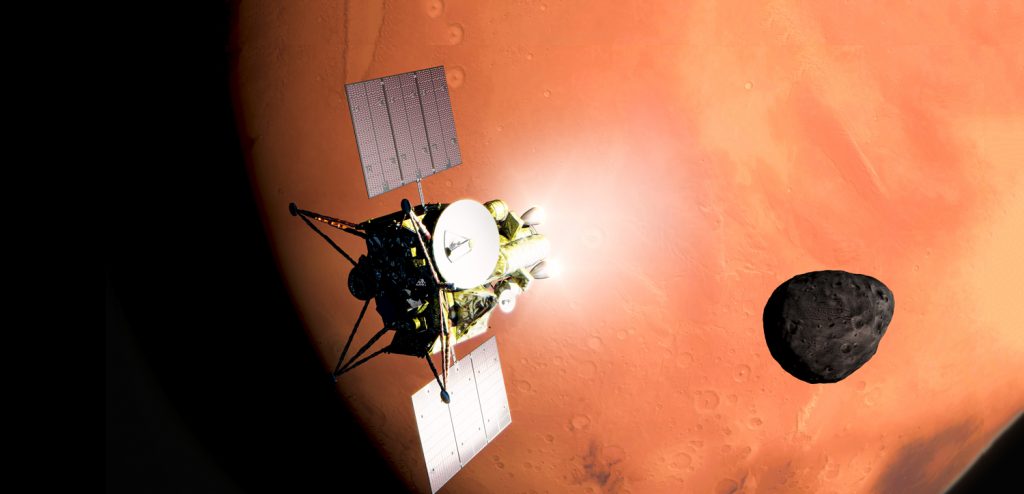
Hyodo emphasises that capture or giant impact scenario, the sample from Phobos will reveal a great deal about how planets form.
“If the capture scenario is correct, we will obtain primitive material that will enhance our understanding of what these consisted of, possibly including the first organics,” he says. “If the giant impact scenario proves correct, we will be gathering a sample from ancient Mars; from the time when the giant impact on Mars occurred.”
It seems a huge amount to learn from such a body as small as a moon.
“With MMX, we will study a tiny moon,” says Hyodo. “But this is not only about the moon, it is also about Solar System material and material from Mars.”
Perhaps surprisingly, the Phobos sample will inevitably contain parts of Mars’s past. This means that regardless of how the moons formed, the sample brought back from MMX will actually be the first Mars sample return.
“Luckily for us, Phobos orbits very close to Mars!” explains Hyodo. “Asteroidal impacts on Mars continuously eject material from everywhere on the planet and this can easily be transferred to the surface of Phobos without strong impact shock damage.”
Martian meteorites collected on Earth are formed from hard, igneous rock as a strong shock-accompanied launch from Mars, the interplanetary journey, and atmospheric entry to Earth destroys anything more delicate. But grains ejected from Mars to land on Phobos have had a much easier launch and ride, and even delicate organics are thought to be able to survive the trip. Even ions from Mars’s ancient atmosphere are thought to have become trapped on the side of Phobos that faces the red planet.
Radioactive elements present in the Martian grains will be able to date the time these grains formed on the surface of Mars. This provides MMX with a unique sample that is collected from all over the Martian surface and dated throughout its history; a veritable log of the planet’s possible habitability and decline. The possibilities for such a collect is one of the reasons why the MMX mission is focussed on the moons rather than the planet itself.

“NASA’s Perseverance will study the Jezero Crater in amazing detail,” says Hyodo. “But the information is limited to Jezero. That might not be typical for Mars’s whole evolution. By contrast, the ejecta collected by MMX will be from everywhere on the surface of Mars without this bias, but at the cost that only a small fraction of the MMX sample will be from Mars. MMX and Perseverance will therefore play the complementary roles of diversity versus detail and together, we can step forward to fully understanding the evolution of Mars.”
Further information: